Abstract
A huge number of parasites can change the behavior of the organisms they infect. In some cases, parasites can even make their hosts display completely new behaviors. We understand very little about the mechanisms and pathways behind this interaction. To learn more, we investigated how a specific fungus changes the behavior of ants, by turning them into “zombie ants”. The infected zombies climb high and latch onto twigs just before they die, which helps the fungus to spread its spores a further distance.
Our results suggest that the fungus takes over the brain, secreting LSD-like compounds, expressing proteins that change serotonin and dopamine levels in the brain, and altering the ability to communicate with nearby ants. Our genome and transcriptome dataset, obtained using both field and lab techniques, allowed us for the first time to dive a bit deeper into the mechanisms used to regulate behavior. In the long run this work might even lead to discoveries related to human brain health.
Introduction
Parasites are organisms that exploit other living beings to further their life cycles. Most of these parasites do so by invading the living system and evolving highly specialized mechanisms for exploiting resources. They can be viruses, microbes, worms and even animals such as insects. Some of these parasites are able to change the behavior of their hosts as part of their strategy. This parasitic trade is a complex result of a very close co-evolution between parasite and host, in which both are in a constant battle with each other to get the upper hand. In some known cases, existing behaviors are slightly changed to serve the parasite’s needs, and in others completely new behaviors are established.
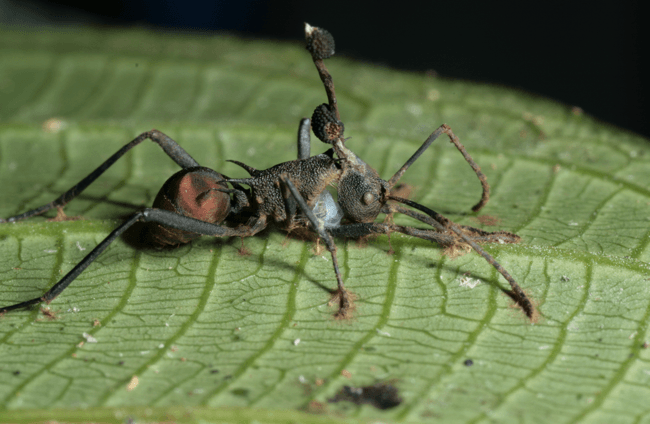
The fungus Ophiocordyceps unilateralis s.l. creates bizarre and beautiful specimens through parasitism.
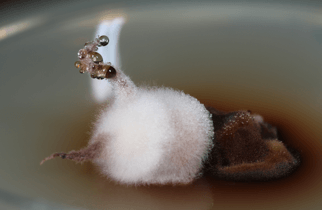
Our project is looking at the phenomenon of manipulated biting behavior we see in the Carpenter ant species Camponotus castaneus that is infected by a behavior manipulating fungus. This is not the only Carpenter ant species that is affected by brain snatchers. In fact, you can find Carpenter ants all over the globe, and as such you can find zombie ants as well.
These fungi manipulate their ant hosts to leave their nest in a drunken zig-zag pattern, until they can climb up vegetation and latch on with their mandibles. After this death grip is established, the fungus then kills the ant using its remaining tissue as a carbon source to grow a structure from which spores are released. Unsuspecting and healthy ants are then infected when they walk across the spores on the ground.
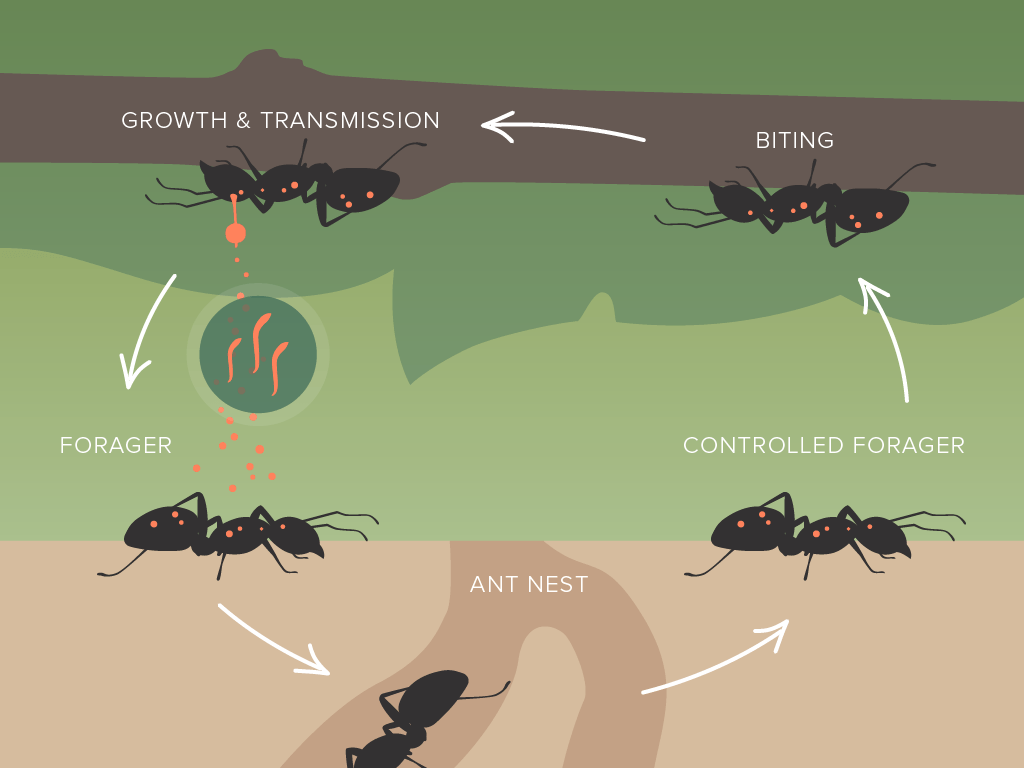
Figure 2: The parasite and host lifecycle.
Ophiocordyceps unilateralis s.l. is the scientific name of the culprit that is able to control the brain of these ants. When it infects the ants, it actually changes the muscle tissue, making it weak so it forces the ant’s mandibles to stay closed after biting itself onto the vegetation. Despite how much is known about the changing ant behavior and the dynamics of this disease, little is known about the diverse molecular mechanisms the fungus uses that lead to this different behavior.
In principle, you can find Ophiocordyceps all over the globe in places where ants are native to the fauna. There are records from all different continents, but the species diversity is higher in the tropical rainforests compared to the temperate woods. One ‘rule’ however appears to be that the woods have to be old, appear fairly untouched, and no landscaping has taken place. Since the forests in Europe at this point are pretty much “man made” we expect that nowadays Ophiocordyceps cannot be found there anymore.
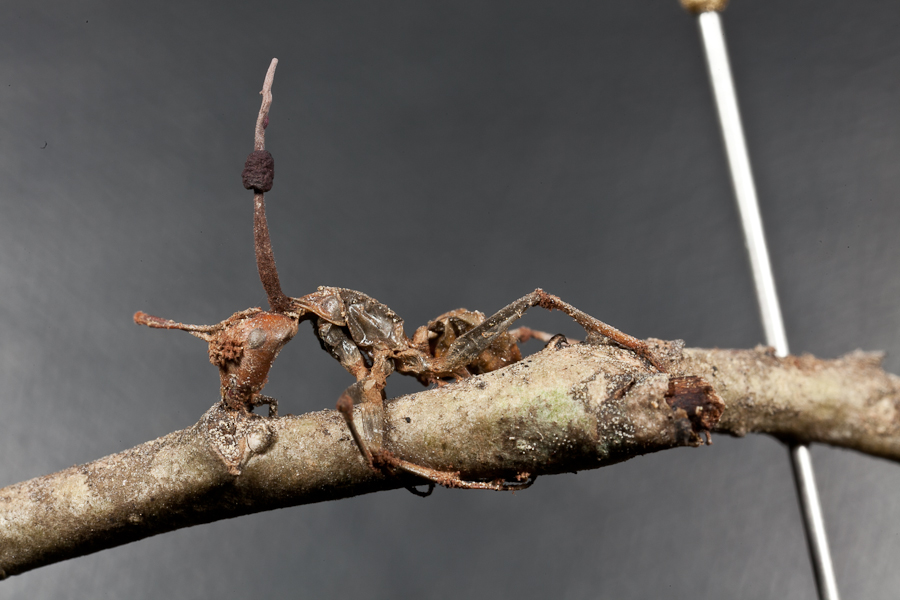
The fungal stalk grows once the parasite has killed the ant.
I became fascinated by fungi when I was a student, and so I decided to do a PhD in fungal genetics. By the end of my PhD I learned through watching BBC’s Planet Earth that certain fungi are able to manipulate insect behavior followed by the growth of beautiful fruiting bodies, leaving behind a rather morbid piece of natural art. The concept of a microbe being able to manipulate an animal’s brain just blew my mind. Ever since, I’ve been working to make the “zombie ants” a model system to study the concept of parasitic behavioral manipulation in detail.
This project was done alongside Dr. Hughes at the Center for Infectious Disease Dynamics, with the Departments of Biology and Entomology at The Pennsylvania State University. The lab is interdisciplinary, combining behavioral ecology, evolutionary biology, entomology, mycology, parasitology and molecular biology. I also collaborated with the genomics and bioinformatics facilities at Penn State, as well as geneticists at Utrecht University in the Netherlands and the Ludwig Maximilian University of Munich in Germany.
Nobody has ever looked into the gene expression of these behavior manipulating fungi infecting ants before, so everything about the project was new. The hypothesis we set off with was to try and focus on the fungal cells right next to the ant brain, at the time the ant was being manipulated and right after the biting ants die. We suspected they would express genes for all kinds of secreted compounds that manipulate the brain, but what exactly and how we didn’t know.
Methods
An important part of this project meant establishing this host-parasite relationship as a model system. This means being able to reliably culture and infect ants, monitor their behavior, and systematically study the molecular mechanism. To be successful, we had to merge field-work techniques with lab manipulations.
Fieldwork
In order to be able to do some controlled experiments in the lab we first had to go out to the field to collect ant colonies. It takes a lot work to dig those up so we spent a few weeks trying to find as many colonies as possible.
The ants used in this study are from South Carolina, and are named Camponotus castaneus. The main reason for our fieldwork to be situated there is a very nice lady called Kim Fleming. In Kim’s free time she likes to go into the woods behind her house for walks and photographing the critters she finds. As such she regularly photographed infected Camponotus castaneus ants biting the trees in her “backyard” and put the pictures up on Flickr. David Hughes found these pictures one day and decided to contact her and go to South Carolina himself to meet Kim and do some sampling. Ever since, Kim is an honorary member of the Hughes Lab and we make a yearly trip to South Carolina to collect ants.
They are mainly active at night. This meant we had to go place bait in the late afternoon to lure the foraging ants. Foraging ants are looking for sugars and protein for themselves and their nest mates so the bait generally consists out of cookies, syrup, and smelly cat food. Then, when it starts to turn dark, we head back to the spots where we placed the bait, hoping there are some ants in the area that found it. We follow those ants back to their nests to mark the entrance. The next day, we would come back to dig up the colony, sort the ants from the dirt, and take them back to the lab.
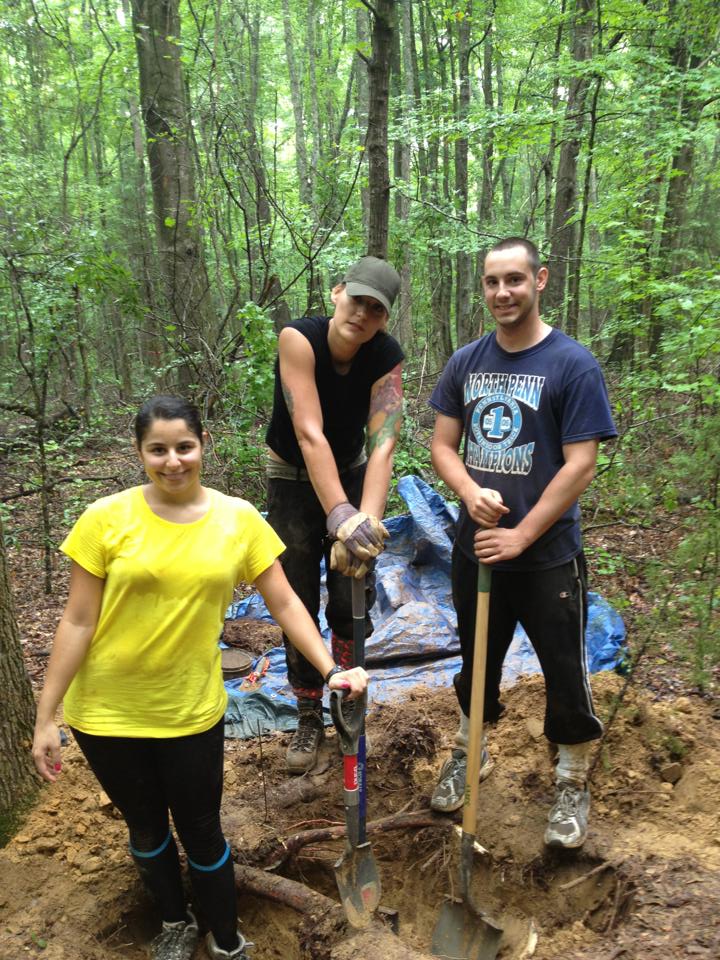
Field work in South Carolina included working day and night to set traps, search, and sort.
Fungal culturing, ant infections and behavioral observations
While in the field, we would try to find samples of ants that had been infected and manipulated by Ophiocordyceps unilateralis, the fungus that creates the "zombies". These dead zombie ants are then used to isolate fungal cultures from. Getting the initial cultures is a challenge because they have to be isolated from ant cadavers collected in the forest that are of course covered in all kinds of microbes. The fungi grow very slowly so it is easy for those other microbes to sneak in and take over. So, whenever I started a new culture I would monitor it closely every day to hopefully save it in time and transfer it to new media. The media I used was regular potato dextrose agar, which is something that is pretty standard in a microbiology lab. The plates are kept under nice warm humid conditions, just the thing that the fungi like.
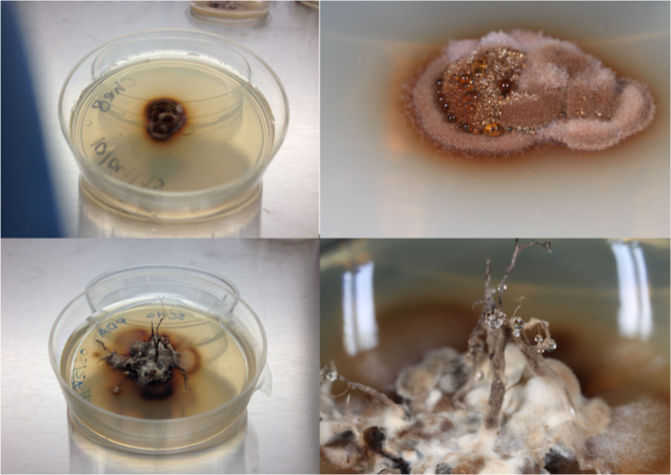
Examples of lab grown cultures from dead ant carcasses.
After the fungus had been isolated and was growing happily, we used it to infect our ants in the lab. This infection is done by injecting the ants with small pieces of fungus. These ants are then individually marked, placed back with their nestmates, and observed for their behavior as the fungal parasite does its business. Once an infected ant is manipulated, it will display the stereotypical biting behavior after they climb onto sticks that we place in the cages.
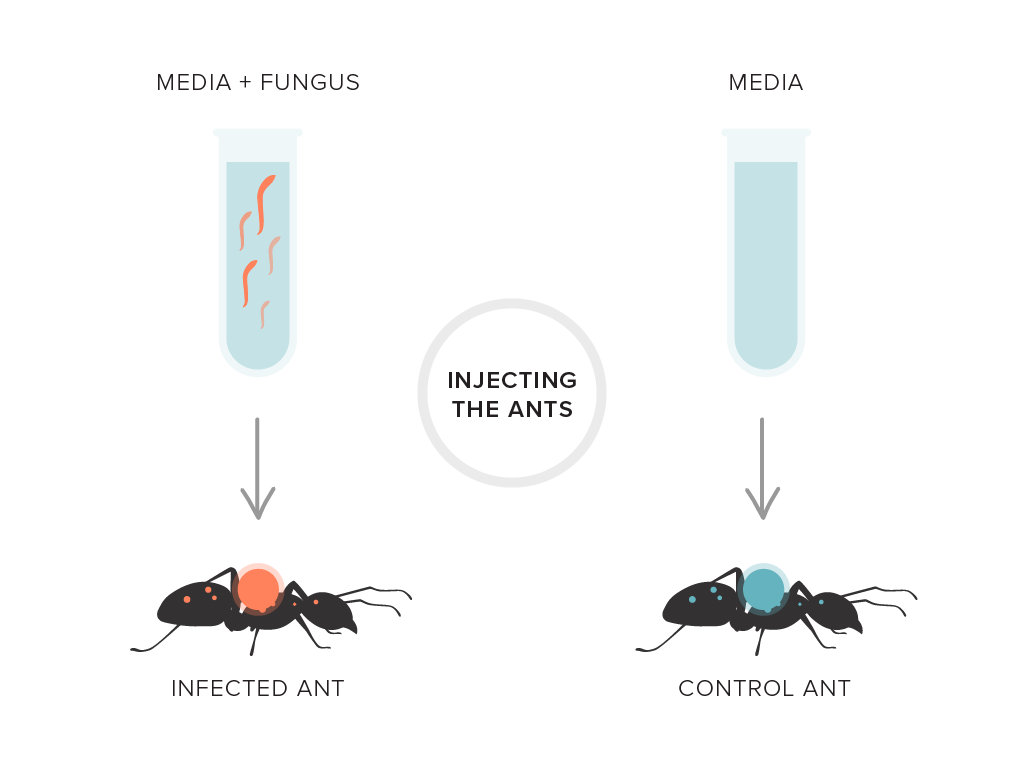
Controlling for infection
To prepare the ants for observation, we also injected ants with media with no fungal parasites for controls
Infecting ants with manipulating Ophiocordyceps fungi under controlled lab conditions is a very new technique. Before this project, this had never been done for behavior manipulating fungi, and especially not followed by looking at the gene expression of parasite and the host at the same time.
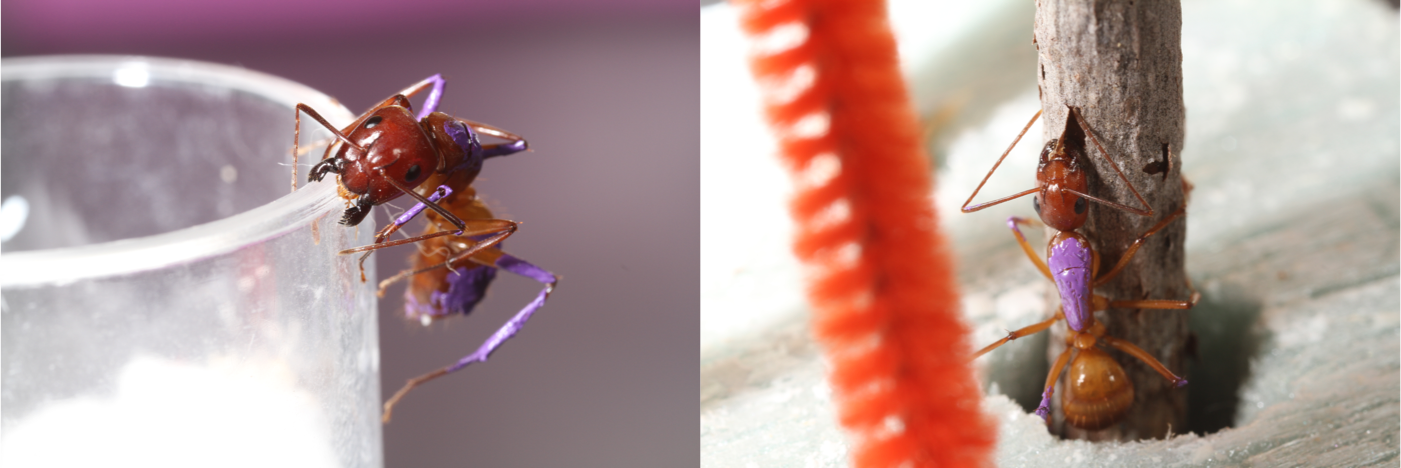
In the lab, we closely monitor each individual ant and mark them by painting the abdomens so we can tell them apart.
Sampling and RNA extraction
To be able to look at the fungal genes involved in establishing manipulated biting behavior, we had to isolate the RNA from the heads of zombie ants, both while they were biting and after they died. The RNA is subsequently translated into proteins, which are involved in all sorts of processes. We need to be able to compare protein expression levels with controls representing non-infected situations to determine if certain genetic pathways are activated or deactivated during biting behavior. For controls, we looked at RNA from healthy ants and the fungus grown outside of the ant host. To make sure we didn’t change the gene expression levels during the sampling, ants were snap-frozen by quickly throwing them in liquid nitrogen and with that preserving the RNA levels.
As part of the sampling we first had the idea to very precisely cut out the fungal cells surrounding the brain, as to only look at those that were interacting directly with it and likely the ones manipulating its behavior. To be able to collect these cells I practiced making very thin (micrometer scale) sections of ant heads, place these sections on a microscope slide and use this slide for laser capture micro dissection. This is a very cool technique in which you use a UV laser placed inside a microscope to be able to cut out cells of interest.
Laser capture microdissection was our first attempt at isolating the RNA in the brains
The technique I chose to make the sections is called cryosectioning. The way it works is that you embed your sample of interest (in this case ant heads) in a resin, which will be completely frozen. Then you take this embedded frozen sample, place it on a stage and start cutting very thin layers with a sharp knife. It is key to find out at what speed to cut, how cold your sample needs to be, what blade to use etc. so your sample stays nicely in one piece. In order to do this correctly you must keep the anatomy of the tissues intact, so I spent a couple of months perfecting the technique. I used healthy ants that had served in other experiments to do this so I could save my infected ant samples for the "real deal".
After a lot of work I was finally ready to test my new skills on infected ant heads. I was very excited, until I started and discovered the fungus attacks the ant’s muscles so harshly that the sections were falling apart. No matter what I tried, I could not keep the tissues intact enough to distinguish between them, making this technique not useful. It is pretty fascinating to find out this parasite does so much damage already while the ants are still alive, but the downside was that I had to change gears.
So instead, we decided to extract RNA from entire ant heads and compare them to heads of healthy ants and the fungus grown outside of the ants. Sounds less fancy than using lasers to cut out cells, but when done correctly it results in a high quality, valuable dataset that gives us information about how the fungus is attacking the muscle tissue as well. So, a little less precise but using this approach, we got to look at how the ant’s gene expression is affected too, instead of only looking at the fungal parasite! So in the end, this worked well for giving us data.
Genomics and mixed transcriptomics
We extracted RNA because this gives us an indication which genes are being actively used during a certain situation. A dataset giving you information about this gene expression is called a transcriptome. For example, if the fungus expresses a certain gene during manipulation that creates a neurochemical signal, but it doesn't use that gene when manipulation has taken place and the ant has died, we will find a lot of RNA corresponding to that gene in the samples that we collected at the time of manipulation and far less in the dead ant samples. When you have samples that contain the gene expression from 2 organisms at the same time (like in our case, we have fungus and ant RNA) we call this a mixed transcriptome.
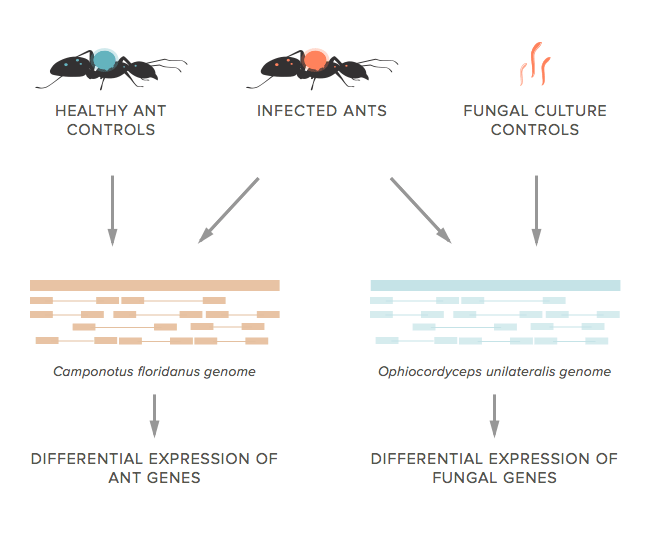
Constructing the transcriptomes involved looking at the puzzle pieces of gene expression from the ant and fungus
We sequenced these RNA samples using a technique called RNASeq. This results in millions of short-length pieces of RNA called ‘reads’. They are like little puzzle pieces that have to be placed in the right order to be able to know what genes they were transcribed from and how high the expression of that gene is. Initially, we tried to solve the puzzle by generating a de novo transcriptome (aka putting the puzzle together without a picture of what it should look like). In the end, no matter what we tried, this resulted in a mess with our reads accidentally mapping to the wrong references, which could lead to drawing the wrong conclusions.
To solve this problem we used the ant and fungal genomes as templates to place our RNA reads in the right order (aka use the genome as an example for how the puzzle should be put together). To be able to do this, we first had to generate a genome for our Ophiocordyceps fungus because it hadn't been sequenced yet. Our Carpenter ant host has also not been sequenced, but another Carpenter ant has been so we used the genome of this related species. This led to the annotation of 7,831 protein-coding genes that were used to help determine the expression levels of the genes in the various RNA samples.
Results
With this project, we produced the first annotated genome of O. unilateralis. In addition, we have also created two entirely new transcriptome datasets, one for the ant host and one for the fungal parasite, which show the expression levels of all the genes found in the genome before, during, and after manipulated biting behavior as seen in zombie ants. These represent the first look into the genetic basis of this phenomenon. Using this new data, we’ve identified which of the genes for both organisms are most likely to be involved in behavioral manipulation.
We did not expect to find just one gene or process to be involved, but instead we anticipated many genes and processes working together. This is indeed our conclusion when looking at our data. In total, we identified 7,831 protein-coding fungal genes. Of these, 498 are significantly more used by the fungus during the manipulated biting behavior when comparing to the other situations in this study. This tells us that they are likely of some importance to changing the ant’s behavior. Here are a few interesting highlights of the genes we have found:
The fungus is producing LSD-like compounds as well as other bioactive secondary metabolites and small proteins, which are affecting serotonin and dopamine levels in the brain.
The fungus is secreting proteins that affect the ant’s locomotion activity
There are proteins that are affecting the ant's ability to communicate through chemosignals with nest mates.
The ant’s biological clock, stress regulation system, and muscles also appear to be affected.
We revealed that the fungal parasite might be regulating immune and neuronal stress responses in the host during manipulated biting, as well as impairing its chemosensory communication and causing apoptosis. Moreover, we found genes up-regulated during manipulation that encode for proteins with effects on behavioral outputs, proteins involved in neuropathologies and proteins involved in the biosynthesis of secondary metabolites such as alkaloids.
One of these alkaloids likely resembles ergot. Ergot was first discovered to be produced by a related fungal plant parasite, from which the drug LSD was originally isolated.
All of our data and results can be found in our August 2015 publication in BMC Genomics. In this paper, our genome and transcriptome data is freely accessible: Link to dataset.
Conclusion
Our big question was to know how certain parasites can bend the behavioral output of the brain to serve their purpose of spreading to future hosts. Learning how parasites like the one in this study can change host behavior so precisely will teach us something about all of these aspects.
What compounds do these parasites use to regulate behavior? And what is changed in a brain of an animal that shows abnormal behavior versus those that act normally? Really, the impact of our project is that it can serve as a stepping-stone for future scientists to answer these questions using the new methods and data we've produced. Zombie ants can become a model system for understanding parasitic behavior change in the brain.
In general, we don’t really know how depressions and anxieties develop and how to effectively help people that have these diseases. Learning how parasites can so precisely tip the scale from normal to abnormal behavior might provide us with some insight. In our study, we for instance find the over-expression of an ant gene in infected brains that likely encodes for a protein that is also found accumulated in brains of Alzheimer patients.
Also, there’s a long track record of fungi producing a wealth of industrially interesting bioactive compounds. The expectation that brain-manipulating species produce novel drug candidates makes genetic studies like this one important from an applied perspective (both in medicine and insect pest control).
At the moment, this study is only providing us with the very first targeted candidates of genes and proteins that likely lead to new behavior. I am continuing with this work by developing forward genetics approaches to study the functions of the genes presented in our results in more detail. For now, I am in Munich, Germany working at the Ludwig-Maximilians-University. You can find me on their website with a description of where I am taking this research in the future.